Building the digital audit and compliance function
EXL helps customer move compliance functions from a sample-based model to a continuous monitoring model. We orchestrate advanced digital interventions to simplify compliance processes, automate tasks, improve reporting, and monitor compliance risks. We drive better outcomes through increased insight into business risks, improved risk forecasting, and stronger assessments of strategic priorities.
What we do
We work with audit and compliance executives to give them increased assurance, deeper real-time insights into deficiencies, early warning signals, and reduce audit fatigue.
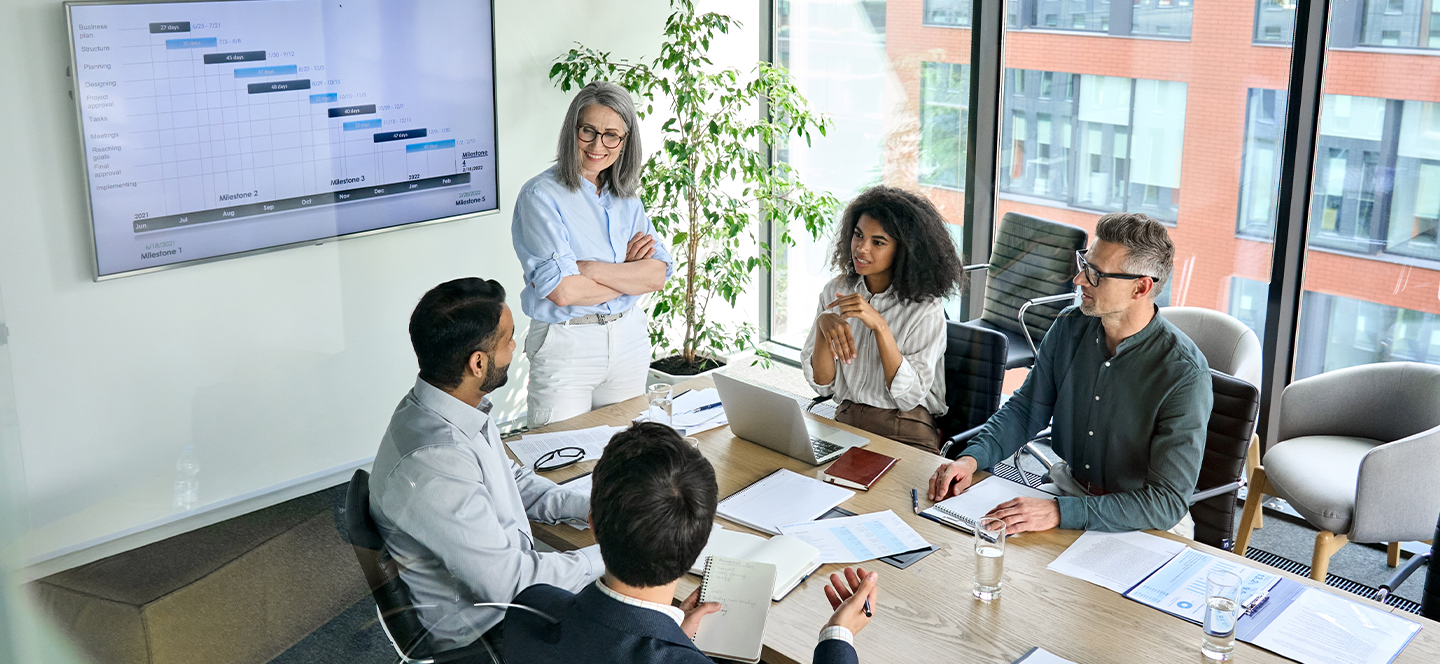
Compliance operations & strategy
<ul><li>Sarbanes-Oxley Compliance</li><li>Internal audit co-sourcing</li><li>Compliance target operating model</li><li>Audit and compliance automation</li></ul>
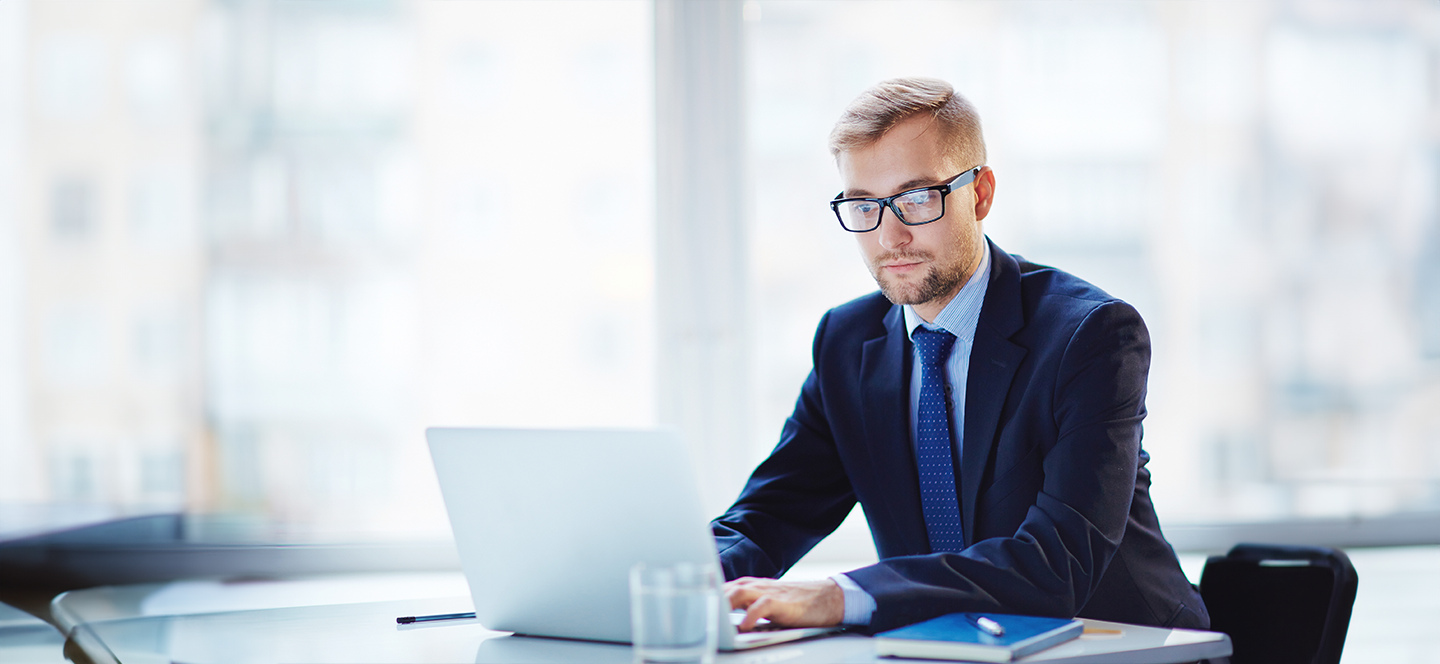
Regulatory change
<ul><li>Data protection and EU-GDPR</li><li>Solvency II, Basel III/BCBS 239</li><li>Insurance Distribution Directive (IDD)</li><li>BREXIT advisory</li><li>MAR 205, HIPAA, AML/KYC, 23 NYCRR 500 (NYDFS), FCPA, PCI DSS, OFAC and others</li></ul>
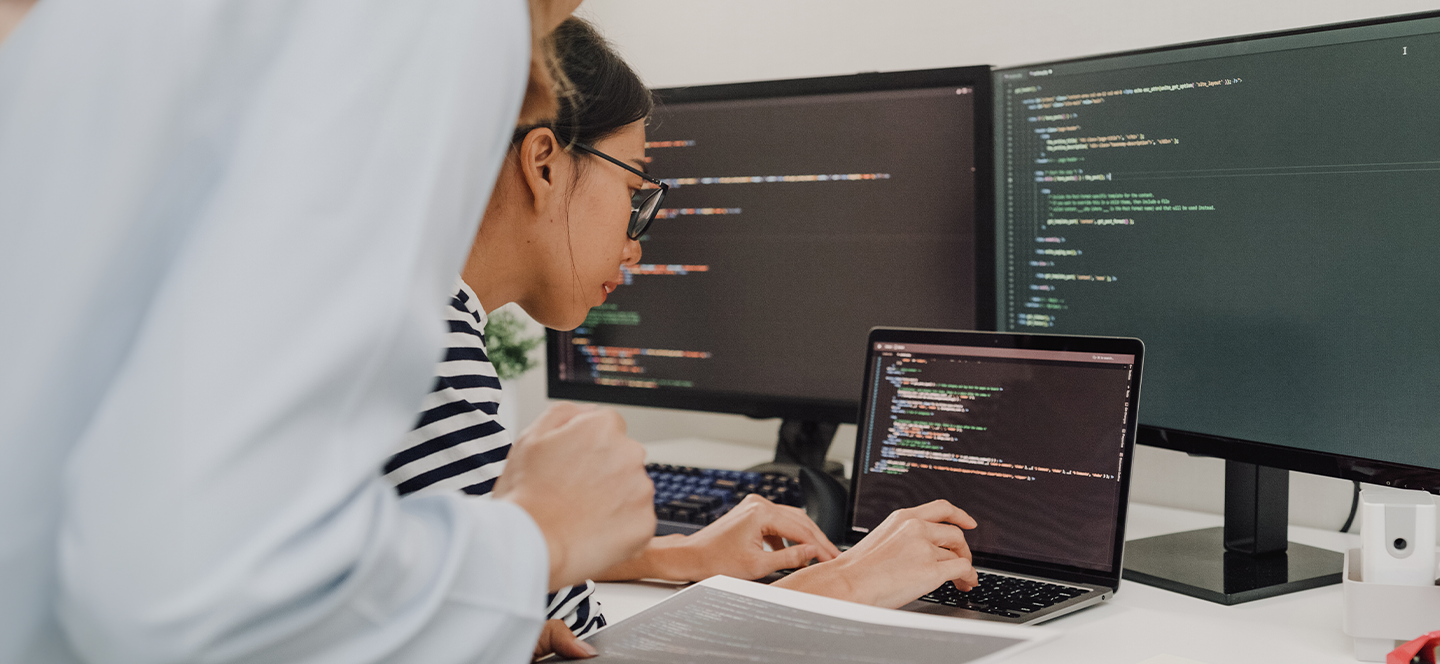
Cyber, fraud & risk
<ul><li>Cyber security audit</li><li>Audit and fraud analytics</li><li>Vendor / third-party risks and controls assessment</li><li>Fraud analytics implementation</li></ul>